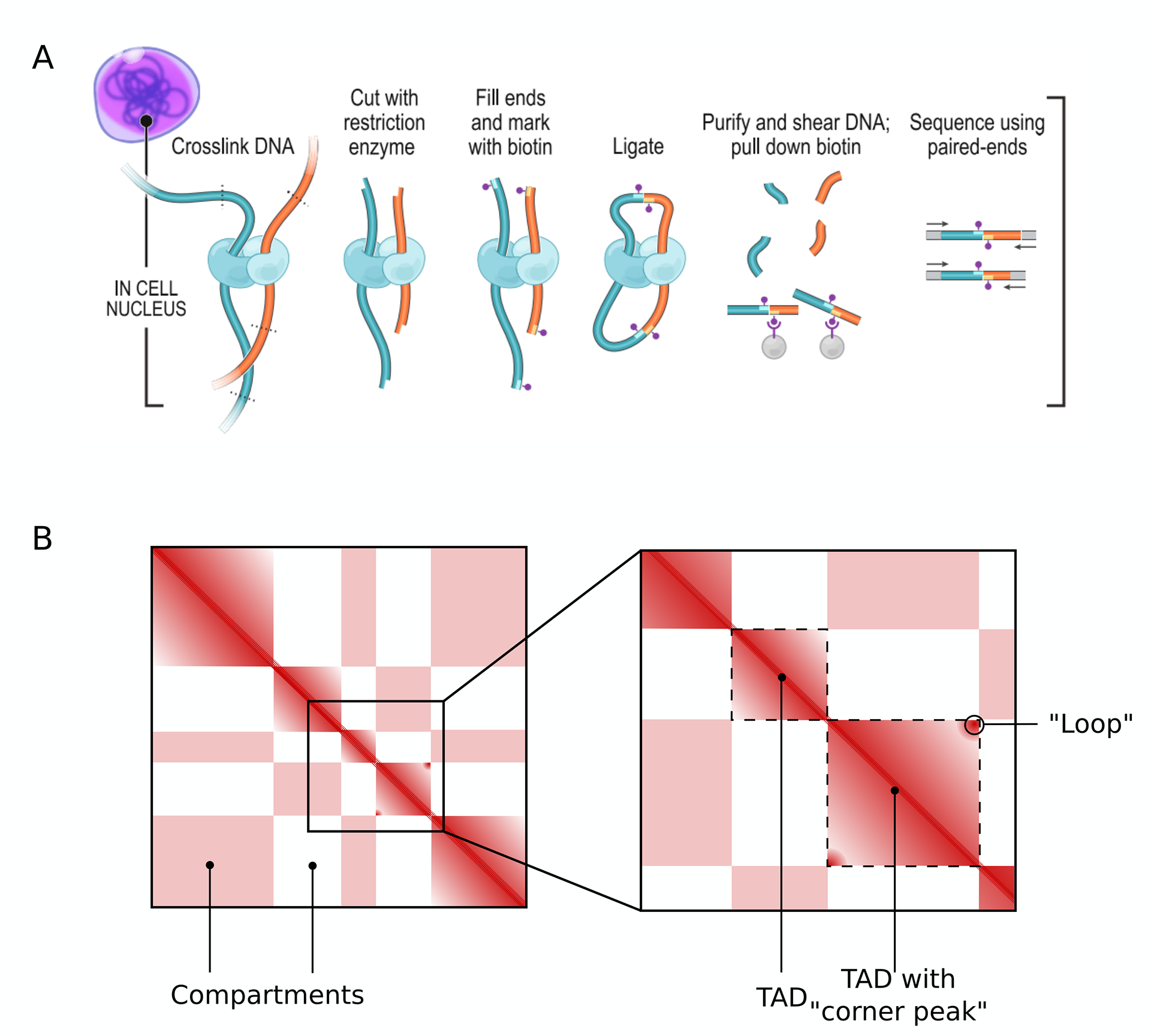
Figure 1: Features of Hi-C contact maps (A) Hi-C technique: nearby DNA segments are cross-linked before digestion, then biotin marks are added. Fragments are ligated, then digested again so that only the DNA fragments resulting from the proximity-based ligation remain. These can then be recovered by pulling down biotin and identified by next generation sequencing [Rao et al., 2014]. (B) In Hi-C contact maps, block squares of enriched contacts along the diagonal are de ned as TADs, alternating regions of enriched and depleted long-range contacts delineate compartments.
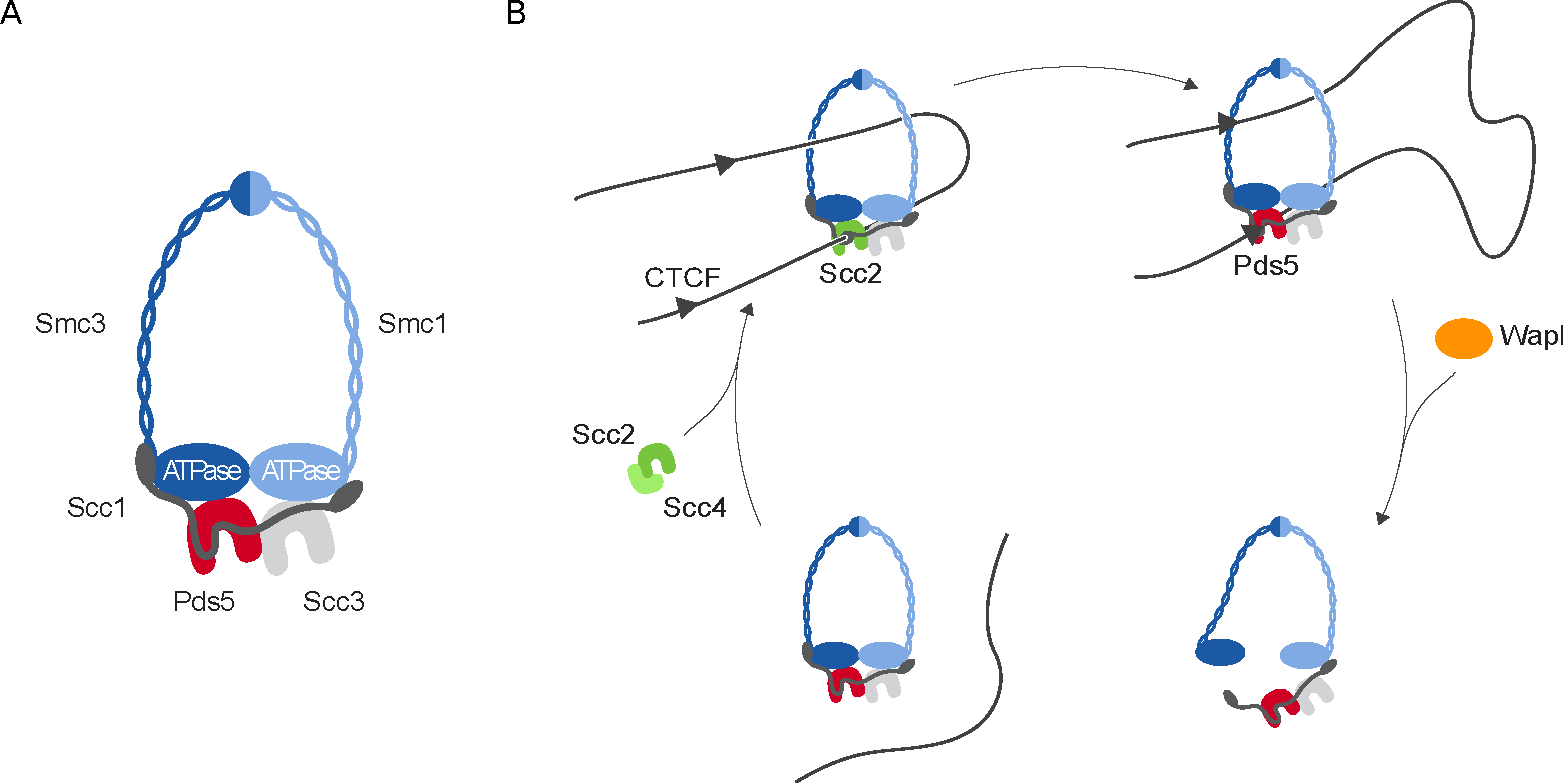
Figure 2: Cohesin complex dynamic interaction with DNA. (A) Cohesin core components (Smc1, Smc3 and Scc1) form a tripartite ring; subunits Pds5 and Scc3 bind to Scc1. Pds5 can be replaced by Scc2 (see panel B). (B) Hypothetical model for loop formation by cohesin: cohesin is loaded onto chromatin by the Scc2/Scc4 loader complex. Loops are enlarged by loop extrusion for which ATP hydrolysis is necessary, which is promoted by Scc2; this process is halted at CTCF sites. Pds5 replacement of Scc2 allows Wapl to open the cohesin ring and chromatin to be released.
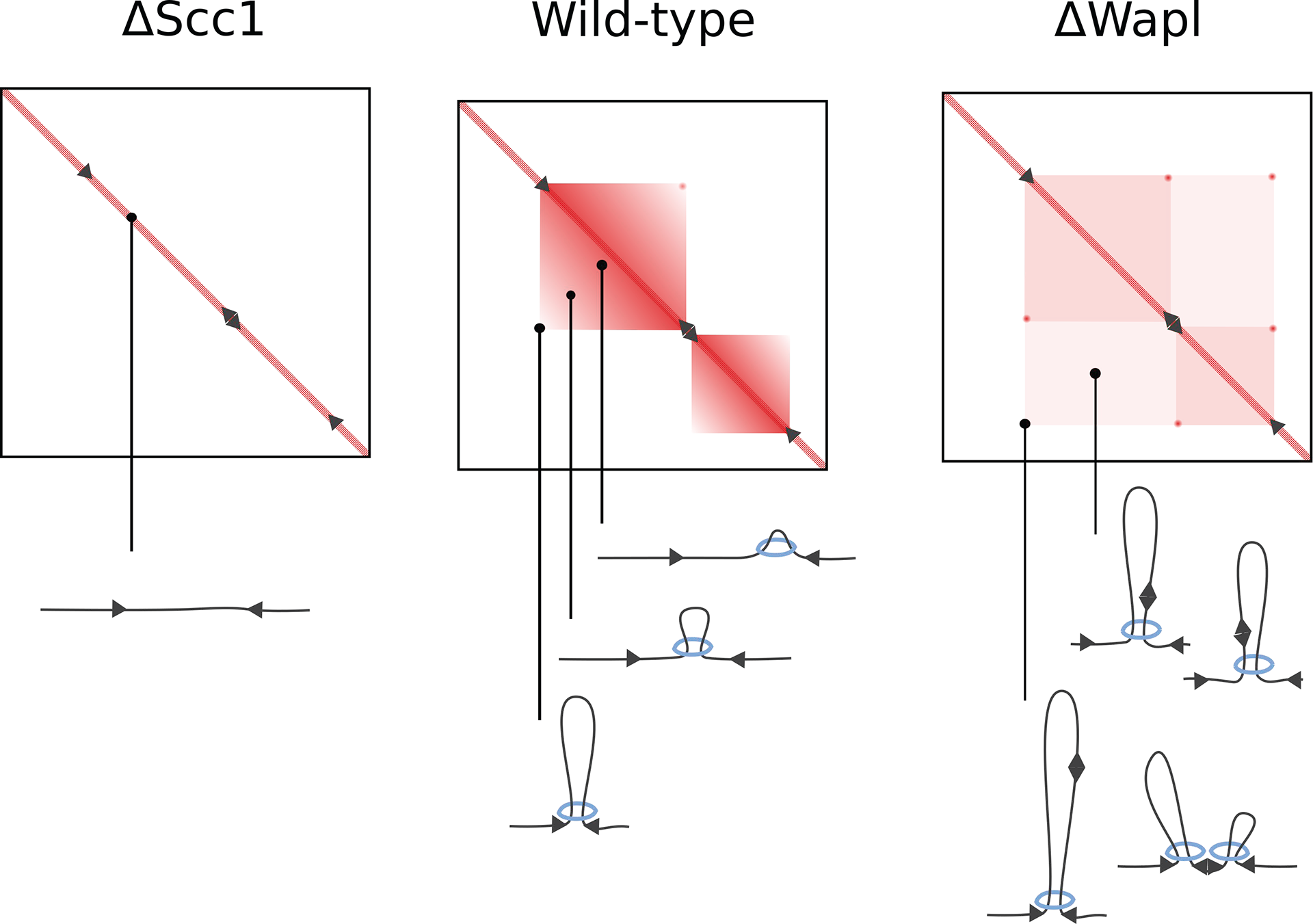
Figure 3: TADs are collections of loops. In cohesin depleted cells (∆Scc1) loops do not form and TADs are lost. Contacts within a TAD can be explained as the collection of contacts formed by cohesin-mediated loops at different moments of their enlargement process. Conversely, in cells with increased cohesin residence time (∆Wapl) this enlargement can continue to form longer loops. As a result, contact enrichment within TADs is decreased, in favour of peaks at CTCF sites (arrows) or where loops collide.
Figure 4: Genome compartmentalization. The plaid pattern seen in Hi-C contact maps reflects the segregation of different genomic segments into compartments A and B.
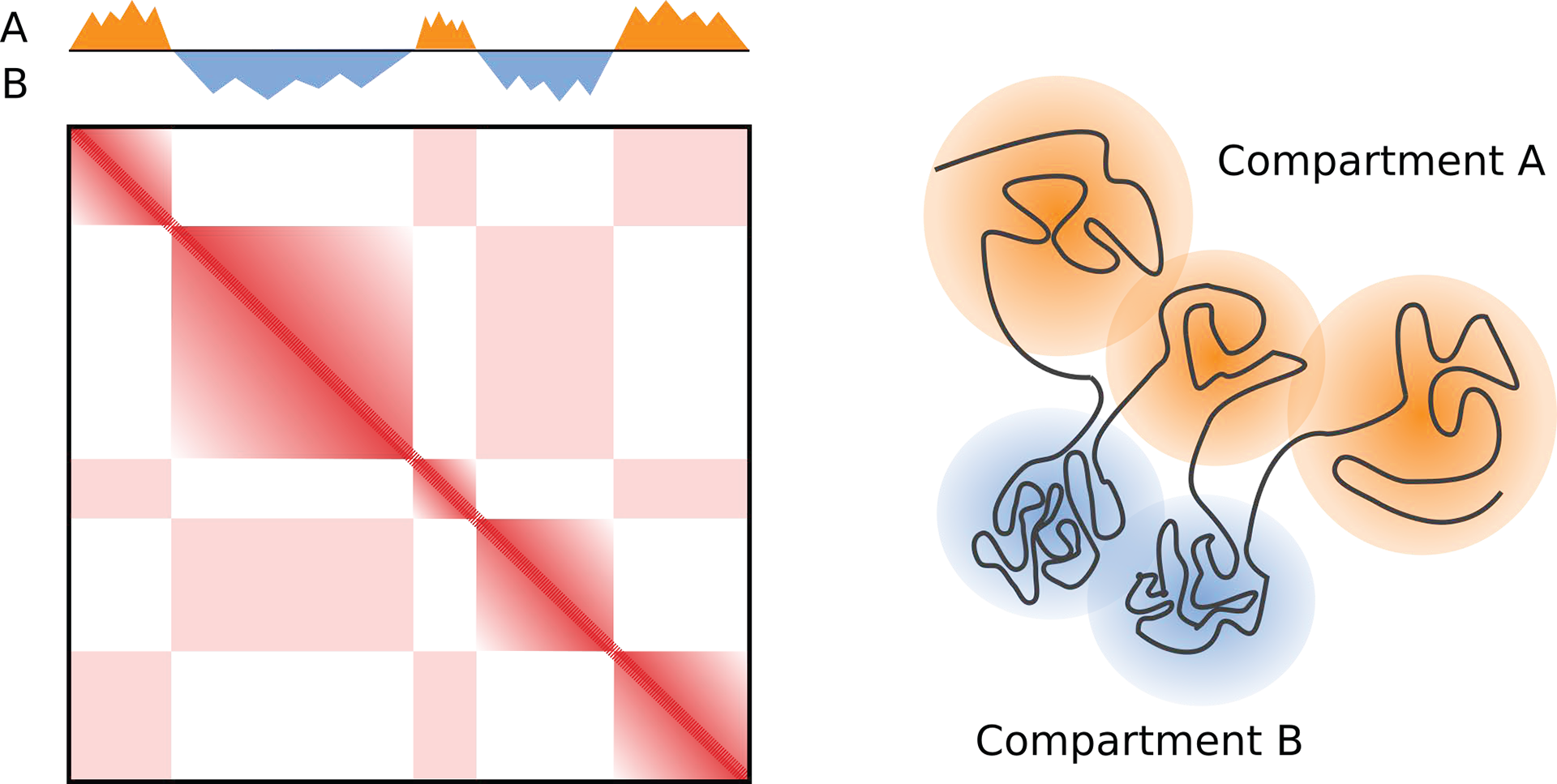
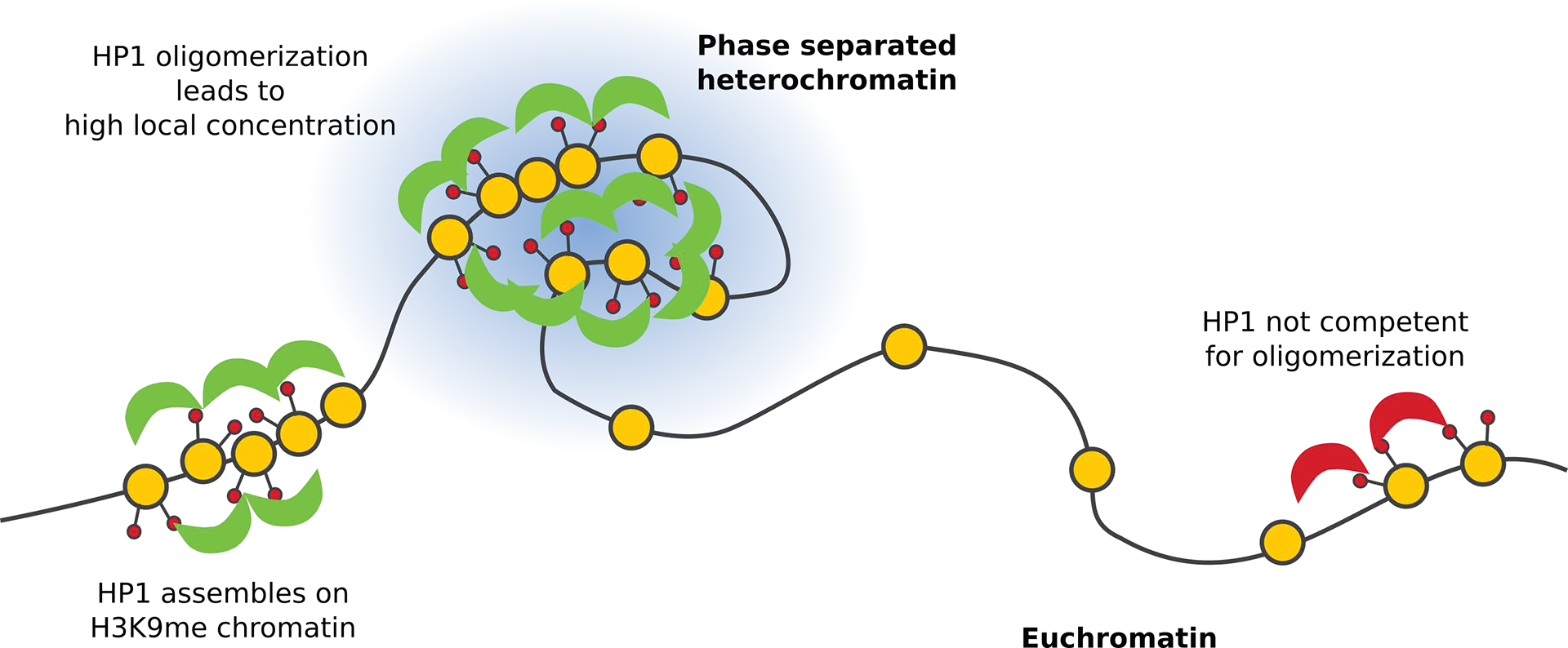
Figure 5: HP1 role in heterochromatin phase separation. HP1 interacts with chromatin with tri-methylated H3K9, commonly associated with heterochromatin. Oligomerization of HP1 increases its local concentration and leads to phase separation of heterochromatin. HP1 not capable of oligomerization does not promote phase separation.
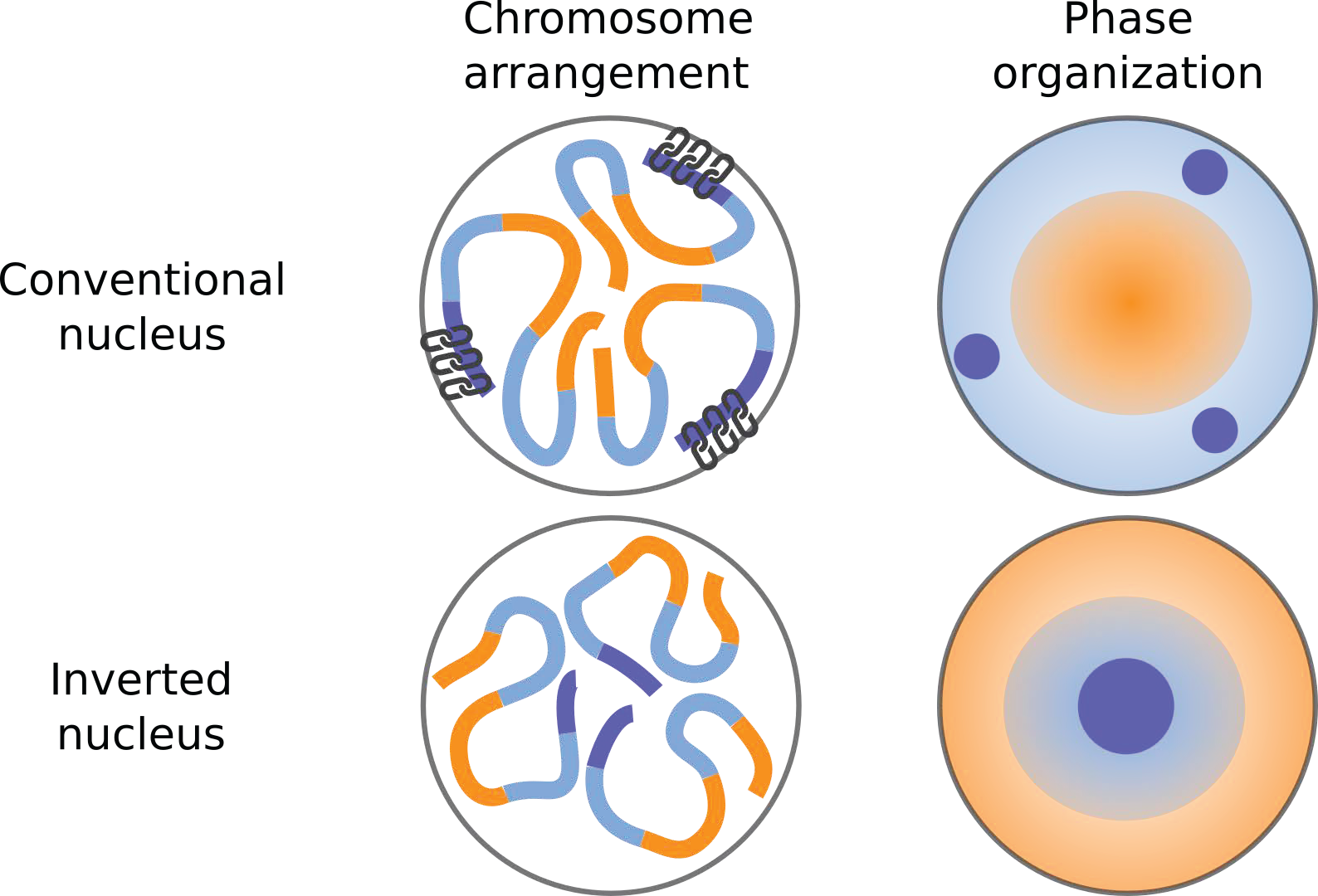
Figure 6: Relevance of interactions with the lamina in chromatin organization. Compartmental segregation of heterochromatin and euchromatin takes place in both conventional and inverted nuclei. Heterochromatin interactions with the lamina result in heterochromatin relocation from the inner nucleus to the conventional perinuclear location.
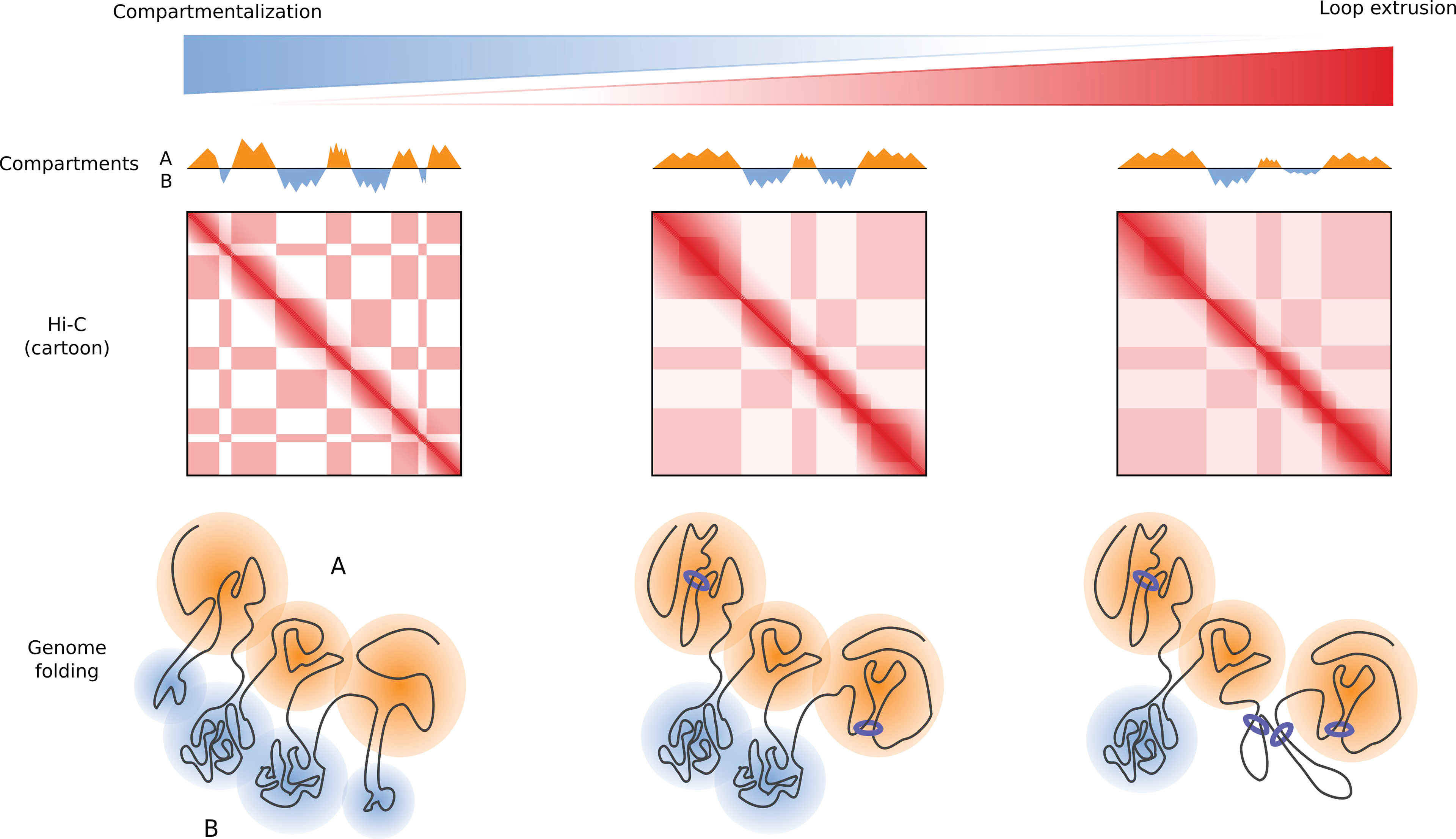
Figure 7: Chromatin looping counteracts compartmental segregation . Lack of loop extrusion reveals ner compartmentalization in the genome. Chromatin loops impede phase separation of small compartments and result in reduced compartmentalization.